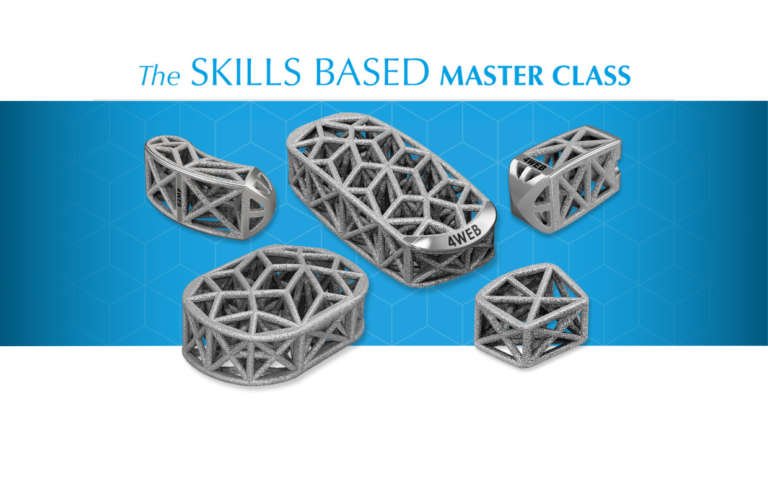
4WEB Medical Clinical Publication | Article 4 of a 4-Part Series
Truss Implant Technology™ for Spinal Interbody Fusion Devices: Delivering Therapeutic Strain to Activate and Amplify Osteogenic Gene Expression
Moving Beyond Surface Technology and into Mechanobiology
Legacy manufacturing technology such as subtractive machining limits the ability to produce spinal interbody fusion devices (IFD) with sophisticated structural designs. Traditional IFD designs feature a ring shape or annular geometry with a large central void. This primitive structural design results in a stiff construct that relegates the implant to being static in nature while the bone works independent of the implant to attempt to fuse over time. Within the past several years development of surface modification techniques such as acid etching has modestly improved the performance of these static spacers. Marketed as “surface technology”, the micro to nano-scale surface features created by this process have been shown to contribute to osteogenic cell differentiation and gene expression.
While surface technology does provide some clinical benefit, these features alone do not improve upon the inadequacies of the static structural design. Through advancements in additive manufacturing, an IFD has been developed with an advanced structural design that capitalizes on well-established engineering principles such as truss architecture, load distribution, and contact mechanics. The proprietary build themes used to produce these truss implants create a hierarchical surface roughness that envelops all of the truss elements or struts throughout the entire construct. The 3D printed truss implant capitalizes on the benefits of surface technology while also taking advantage of the structural mechanics of the truss design. Under physiologic load, the truss implant delivers therapeutic strain to the surgical site that stimulates a mechanobiologic response in adjacent cellular material, further enhancing osteogenic activity and accelerating the fusion process.
4WEB Medical’s Truss Implant TechnologyTM (4WEB Medical, Frisco, TX, USA) is an open architecture design that consists of an empirically derived interconnected web of truss elements called struts. The truss implant functions as a high-strength, lightweight structure with a significant amount of open space to accommodate bone growth throughout the entire construct.
Produced with electron beam melting (EBM) technology, the additively manufactured truss implant consists of a hierarchical surface roughness with features that span from the macro- to nano-scale (Fig. 1). These surface features resemble the dimensions and morphology of resorption lacunae produced by osteoclastic activity. By mimicking the surface characteristics of native trabecular bone, the truss implant’s surface technology provides an osteogenic stimulatory effect which supports local bone growth factor expression, direct osteoid deposition, and bone formation.
Beyond surface technology, the truss implant capitalizes on an architecture that distributes physiologic load through its struts. Experimental ex vivo evidence has verified that under physiologic loading conditions, the 4WEB truss implant distributes compressive and tensile strains through its struts which can stimulate osteogenic activity.
Truss Implant TechnologyTM provides a synergistic ecosystem between surface technology and the mechanics of the macro truss design to provide a cascade of stimulatory effects on adjacent cellular material. Specifically, it is believed that the surface technology initiates osteogenic differentiation and then the truss design amplifies osteogenic activity via a mechanobiologic mechanism.
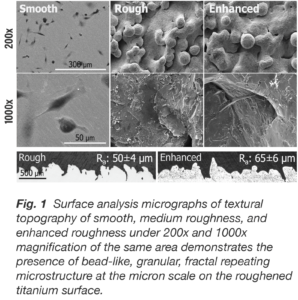
A two-phase study was conducted to determine:
1) Whether 4WEB’s proprietary 3D printed surface technology, when exposed to a culture of hMSCs, would stimulate an osteogenic response by evaluating hMSC morphology, adhesion, clustering, and osteogenic differentiation, and
2) To quantify the impact of delivering strain to adjacent cellular material by measuring osteogenic gene expression of hMSCs subjected to cyclic loading and comparing the results to the baseline osteogenic response gathered from the hMSCs seeded on the static surface technology.
When passively exposed to three titanium surfaces, cultures of hMSCs responded in distinctively different fashion both qualitatively and quantitatively with respect to cell morphology. By day 14, cells on the smooth surface are flat and spread continuously and uniformly, whereas on both roughened titanium surfaces, the cells are clustered within the crevices and interstices similar to the 3D porous structure of trabecular bone.
To characterize gene expression by surface type, hMSC osteogenic differentiation was evaluated by reverse transcription-polymerase chain reaction (RT-PCR) for Type-1 collagen and osteocalcin. hMSCs were cultured in basal growth media. When cultured in basal media, average collagen expression at day 14 was markedly lower (p<0.05) for both roughened surfaces compared to the smooth surface. In contrast, average osteocalcin values were significantly higher (p<0.05) for the roughened titanium surfaces compared to the smooth surface (Fig. 2). In conjunction with the cell morphology showing flat, widely spread cells associated with smooth titanium and marked cell clumping with roughened surfaces, these gene expression results reinforce a greater potential for fibroblastic lineage differentiation for smooth surfaces and osteogenic differentiation for roughened surfaces.
To assess the impact strain provided by the mechanics of the truss implant architecture, finite element modeling was used to estimate the load necessary to create the surface strain in the range to support osteogenesis. To confirm that the strain would not negatively impact the cells or cause cell death, dynamic 3-point bending load was applied to the truss element with a displacement of 0.35 mm for three hours and compared to a non-loaded control with no discernible change found in the living cell population after loading.
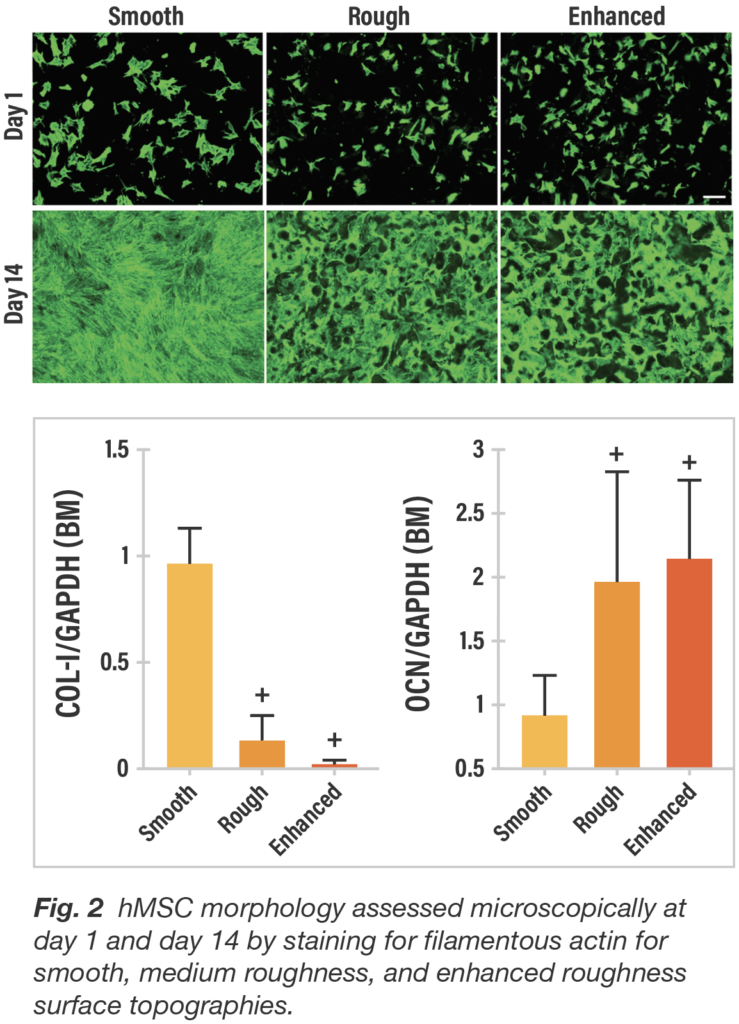
This study corroborates and refines previous research demonstrating that a roughened titanium surface provides a more compatible substrate for osteogenesis than a smooth titanium surface. It was found that the surface with enhanced roughness (the surface found on 4WEB truss implants) offered the best micro-environment for predisposing hMSCs toward an osteogenic lineage. This finding suggests that the surface features on the implant created by additive manufacturing are bioactive with respect to the ability to facilitate de novo bone formation and provides a similar stimulus to adjacent cells as implants treated with acid etching and other subtractive techniques.
The study also showed that dynamic loading significantly increased osteogenic gene expression compared to static implants with surface technology alone. The findings suggest that the 4WEB implant surface features work synergistically with the truss architecture. The surface technology initiates osteogenic differentiation and then the truss design amplifies osteogenic activity via a mechanobiologic mechanism. Truss Implant TechnologyTM actively participate in the fusion process giving patients the best chance to achieve a fully integrated, mechanically-solid, endplate-to-endplate arthrodesis.
The transition from static implants to active implants that respond to load and communicate with the surrounding biology through kinetic mechanisms will provide a pathway to develop personalized implants that are programmed to behave a very specific way based on design inputs such as such as the patient’s weight, bone density, and anatomical location leading to optimization strategies that will improve patient outcomes.
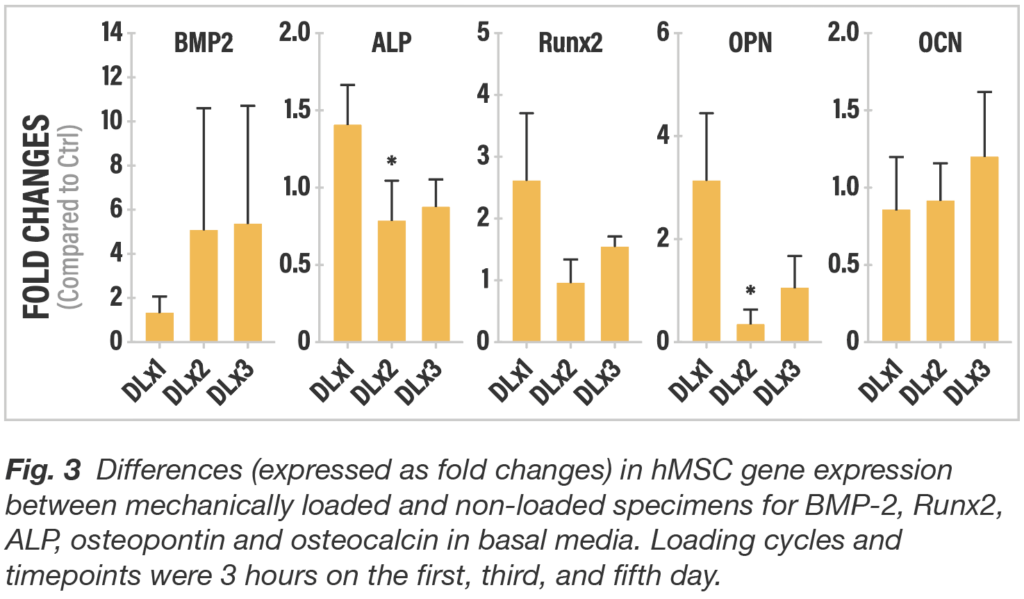
Achieving amplified osteogenic gene expression through a synergistic relationship between surface technology and the mechanics of the macro truss design confirms that Truss Implant TechnologyTM provides a platform to design and develop implants that provide a cascade of stimulatory effects which accelerate the fusion process and improve clinical outcomes
Data on file at the University of Pennsylvania Department of Orthopaedic Surgery and Bioengineering, 4WEB Medical